Data transfer between unmanned systems and ground control
stations is a challenge for any unmanned system. Data transfer between an
unmanned system and a ground control station which are planets apart becomes
even more of challenge given the limitations of our current technology.
Understanding the methods behind the Mars Curiosity Rover communications system
will help us gain understanding about data management, treatment, and movement
in any unmanned system. Additionally, being able to understand the onboard
sensor and how they interact with the data transfer architecture in terms of
power requirements, data storage requirements, and data treatment methods, will
help us analyze the current state of technology. Upon a detailed study of the current
situation, proposed future changes could streamline the entire data transfer
process from the sensor to the final product.
Data Format, Protocol, and Storage
The Curiosity Rover has a network of satellites that
allows the rover on the surface of Mars to communicate with the ground control
station (GCS) on earth at high data rates. Unlike smaller unmanned systems,
direct communication between the ground station and the vehicle are
impracticable due to the power requirements and bandwidth limitations that
exits when transmitting data between Earth and Mars. The rover and the GCS can
be as far as 400,000,000 km apart, and to transmit data over that distance
while using a relatively feasible mount of power, the bandwidth peaks at 800 b/
s using the onboard high gain antenna, which utilizes X band frequencies (Gordon,
2012). This type of communication can transfer telemetry and navigational commands
to the rover, but sending raw imagery or data from one of its many sensors
would be extremely impractical. For large data the Curiosity Rover utilizes the
Mars Reconnaissance Orbiter (MRO) to relay its data back to earth. The MRO
orbits only 275km over the surface of Mars, which exponentially decreases the
power requirement to transmit large data files off the surface of Mars (Taylor,
2006). The orbiter is designed not only to relay and amplify signals back to Earth,
but it is an instrument of science loaded with its own sensors that are used in
conjunction with the rover. The final piece of the communications architecture
is the GCS. There are three large ground stations, one in Goldstone,
California, one in Madrid, Spain, and one in Canberra, Australia. These GCSs
are on a much larger scale than any terrestrial based unmanned system GCS. They
come standard with one 70m parabolic antenna and at least two 34m parabolic
antennas. By creating huge power hungry antennas on the earth, the size and
power required by the orbiter and rover to transmit and receive data can be
decreased (Gordon, 2012).
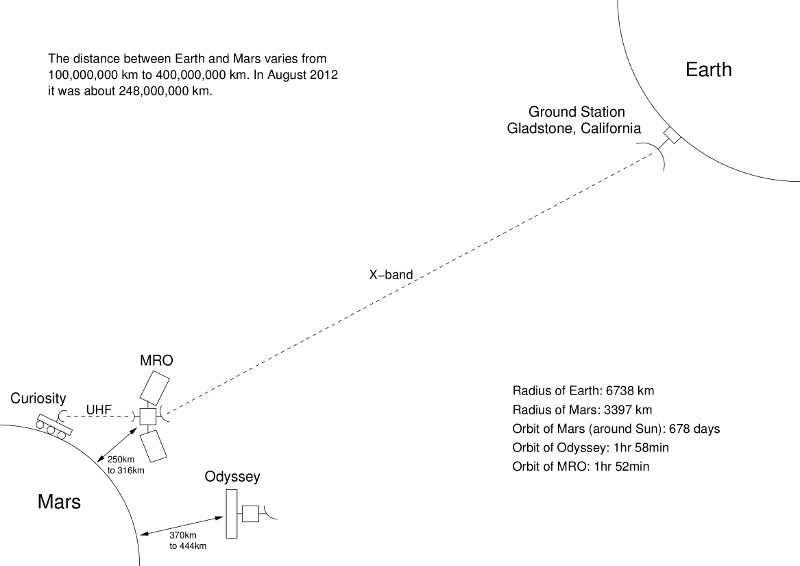
Onboard Sensors
In terms of sensors onboard Curiosity, there are many high
tech sensors that have a range of purposes (NASA, 2015). In an effort to study
data transfer techniques; analyzing the sensors with the highest data transfer
requirement will assist in understanding how to streamline the process in the
future. Some of the highest data producing sensors on the rover are the mast cameras.
There are two 2-megapixl cameras that not only capture imagery, but can be used
as a stereo pair in order to conduct 3D mapping of the rovers immediate
surrounds. Anyone with a background in photography would think 2MP cameras are
not the best choice for a 2.5 billion dollar space probe, but this is the first
step of the data treatment method utilized by the engineers on the rover
project. By utilizing a lower megapixel camera, the data transfer requirement
is decreased before utilizing compression, or other forms of data treatment.
The mast cameras utilizes a 1600 x 1200 pixel resolution interline camera
sensor (Cangeloso, 2012). This camera is capable of storing up to 5500 full
size images on the actual cameras memory itself, which acts as a buffer. This capture
resolution roughly translates to about a 1.4mb image file in its raw form
(Gordon, 2012). This is where the use of the MRO and UFH radio communication
come in. The UHF radio on the rover can pump out much higher bandwidth, which
is up to 256 kb/s. This is done with a 12 watts transceiver. If the rover was
required to send via its high gain antenna straight back to Earth via X-band
communications, data transfer could only top out around 800 b/s and required 15
watts of power. This would mean the same images would take much longer to
transmit and require the 15watt transceiver to be active and drawing energy for
a much longer period of time (Taylor, 2006). The power and time constraints
placed on the entire system when the MRO is not used would severely limit the
mission success of the rover.
Alternative Data Treatment Strategy
Since 2004, which is when Curiosity and the MRO were
designed many things have changed in terms of image compression and data
storage. Starting with the sensor, I would recommend the use of a higher
resolution camera pair, but include the use of lossy image compression. This
would have two aspects, it would increase the native resolution and quality of
the imagery coming from the mast camera set, but the lossy compression would
reduce the data back into a manageable data set that would be easily relayed
back to earth (Chin, 2013). The second methodology in terms of data transfer
would be increasing the onboard storage capacity from about 8 GB to 100 GB per
sensor. Along with the increased storage, I would implement a server based
tagging method to the imagery that would add metadata and allow scientist to
query the server and then pull full resolution imagery as needed. Not having to
send back all the imagery at full resolution would save bandwidth to allow
simultaneous transfer of the “current view” low resolution imagery as well a
full resolution stills that are being queried by scientists for further
investigation.
Both data storage and processing power are increasing
exponentially every day. The physics of data transfer and the power required to
accomplish it are not as rapidly advancing as fast, and this is why
alternatives and advances in data transfer should focus on the digit aspects
rather than the electromagnetic aspects. Long distance laser data transfer may
be a long term solution to the current slower moving portions of the
electromagnetic spectrum that we utilize, but with todays rapid increase in
processing power, the near term solution may be in compression and processing
advances in data transfer.
References
Gordon, S. (2012, January 1).
Talking to Martians: Communications with Mars Curiosity Rover. Retrieved
February 8, 2015, from https://sandilands.info/sgordon/communications-with-mars-curiosity
Taylor, J. (2006). Mars
Reconnaissance Orbiter Telecommunications. DESCANSO Design and Performance
Summary Series, (Article 12). Retrieved February 8, 2015, from http://descanso.jpl.nasa.gov/DPSummary/MRO_092106.pdf
Makovsky, A. (2009). Mars Science
Laboratory Telecommunications System Design. DESCANSO Design and Performance
Summary Series, (Article 14). Retrieved February 8, 2015, from
http://descanso.jpl.nasa.gov/DPSummary/Descanso14_MSL_Telecom.pdf
NASA. (n.d.). Mars Science
Laboratory; Curiosity Rover. Retrieved February 8, 2015, from http://mars.nasa.gov/msl/
Cangeloso, S. (2012, August 9). Why
does the $2.5 billion Curiosity use a 2-megapixel camera? | ExtremeTech.
Retrieved February 8, 2015, from http://www.extremetech.com/extreme/134239-why-does-the-2-5-billion-curiosity-use-a-2-megapixel-camera
Chin, M. (2013, December 18). New
data compression method reduces big-data bottleneck; outperforms, enhances
JPEG. Retrieved February 8, 2015, from
http://newsroom.ucla.edu/releases/ucla-research-team-invents-new-249693
No comments:
Post a Comment